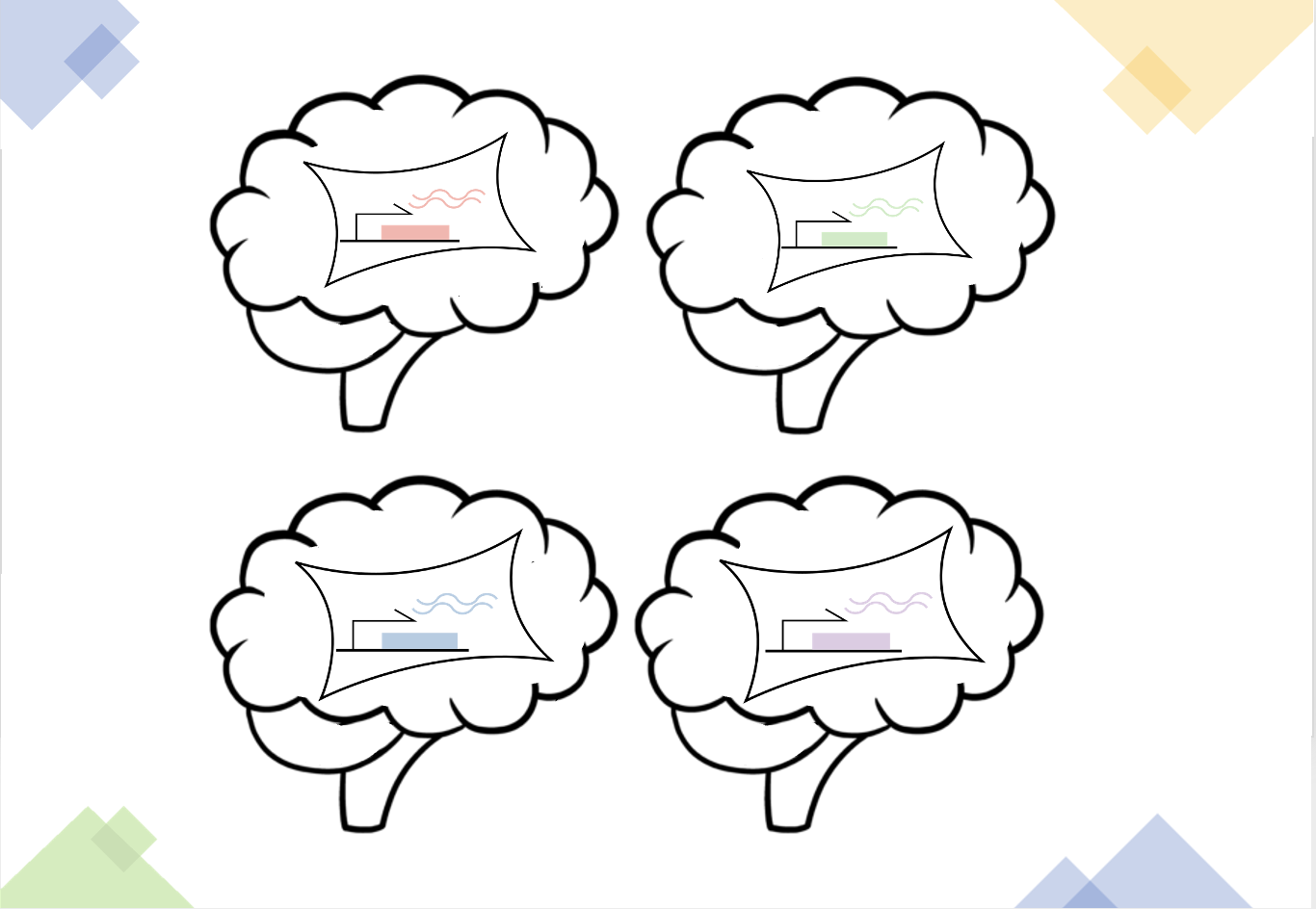
Harnessing Cellular Intelligence for Drug Discovery
Harnessing Cellular Intelligence for Drug Discovery
This is part one of our four part series on Octant's multiplexing capabilities. To read more on multiplexing for drug discovery, check out our posts on broad target scans, reporter development, and deep mutational scans.
It’s no wonder that screening campaigns in living cells can dramatically improve the early drug discovery process given so many diseases involve complex changes in cellular behavior that are difficult to faithfully reproduce in biochemical and biophysical assays. Despite its ability to offer unique insights into mechanisms that drive disease, high-throughput cellular screening is still underutilized in industry because it’s hard to build, optimize, and scale. We’re hard at work solving those problems. We’ve built advanced tools which enable us to interrogate disease-relevant cellular mechanisms en masse using our Octant Navigator platform. The Octant Navigator advances drug discovery by driving high throughput chemistries through engineered cellular systems, which we’ve been able to scale up in large part due to our multiplexing capabilities.
In this four-part blog series we’ll share a high-level overview of how we use multiplexing to enable Octant’s “Cellular Intelligence” approaches. Specifically, we’ll discuss how we apply multiplexing for assay optimization, broad target screening, and deep mutational scanning. We’ll lay out some basic explanations for anybody interested in how synthetic biology can be used as a tool for drug discovery, and also explore some of the technologies deeper for the bioengineers out there.
Using Nucleotide Barcodes to Multiplex Biological Data
Multiplexing to increase data throughput dates back to the 1800s when it was developed to improve telecommunications and electronics. It has also been used to enrich biological outputs. For example, multicolor flow cytometry and microscopy use it by coupling biological outputs with proteins that emit different colored wavelengths, but that has been limited by a small handful of color ranges.
At Octant, we employ short strings of nucleotide sequences (we call them "barcodes") to tag and identify the outputs of cellular processes. Using nucleotide barcodes massively increases the multiplexable outputs of an assay. A string of 20 nucleotides has 420 (over a trillion) unique combinations readable by a sequencer. This scale completely changes the kinds of questions one can ask in biology, which is why we’ve spent over a decade innovating in this space. The next few sections walk through the nature of questions that multiplexing can help answer in a bit more detail.
Multiplexing For Rapid High-Quality Assay Development
Multiplexed assays are a game-changing way to rapidly build and de-risk cellular assay systems at a new scale. Building faithful cell models requires optimizing hundreds of variables from gene circuit architectures to assay conditions (for example, genetic variables like promoters and response elements to environmental variables like time and treatment conditions). It would be prohibitively time-consuming, expensive, and result in poorly controlled data to manually build and test thousands of possible combinations. By multiplexing these assays we can run optimizations in carefully controlled conditions to enable a pace and quality of assay development that would have felt like magic just a few years ago.
Once we’ve optimized across thousands of possibilities to arrive at cellular models that read out on specific aspects of cellular activity, we can bring those assays together to build a more thorough understanding of what’s happening in complex cellular environments.
Multiplexing To Engineer Fine-Tuned Pharmacology
Humans have been ingesting drugs that modulate complex biological processes since ancient medicine. Many of these molecules found in nature exerted therapeutic effects by acting on multiple biological targets. A classic example of this is salicylic acid, derived from willow bark, which binds to many receptors that make it a potent anti-inflammatory compound with cardioprotective and antitumorigenic benefits.1 Known commonly today as aspirin, a derivative of this ancient wonder-drug elicits therapeutic effects by acting on cyclooxygenases, tumor necrosis factors, and kinases.1 The industry refers to this phenomenon of hitting many target as polypharmacology.
Although serendipitous, Aspirin’s polypharmacology is what drives its impact and now more and more companies are trying to intentionally engineer drugs to act on multiple targets. One current example of polypharmacology in the clinic is Eli Lilly’s investigational drug Retatrutide, which acts as a triple agonist against glucagon, GIP, and GLP-1 receptors (Figure 1), engendering even greater weight loss in clinical trials than approved blockbuster drugs Semaglutide (GLP1-R agonist) and Tirzepatide (dual GLP1-R/GIP agonist).2
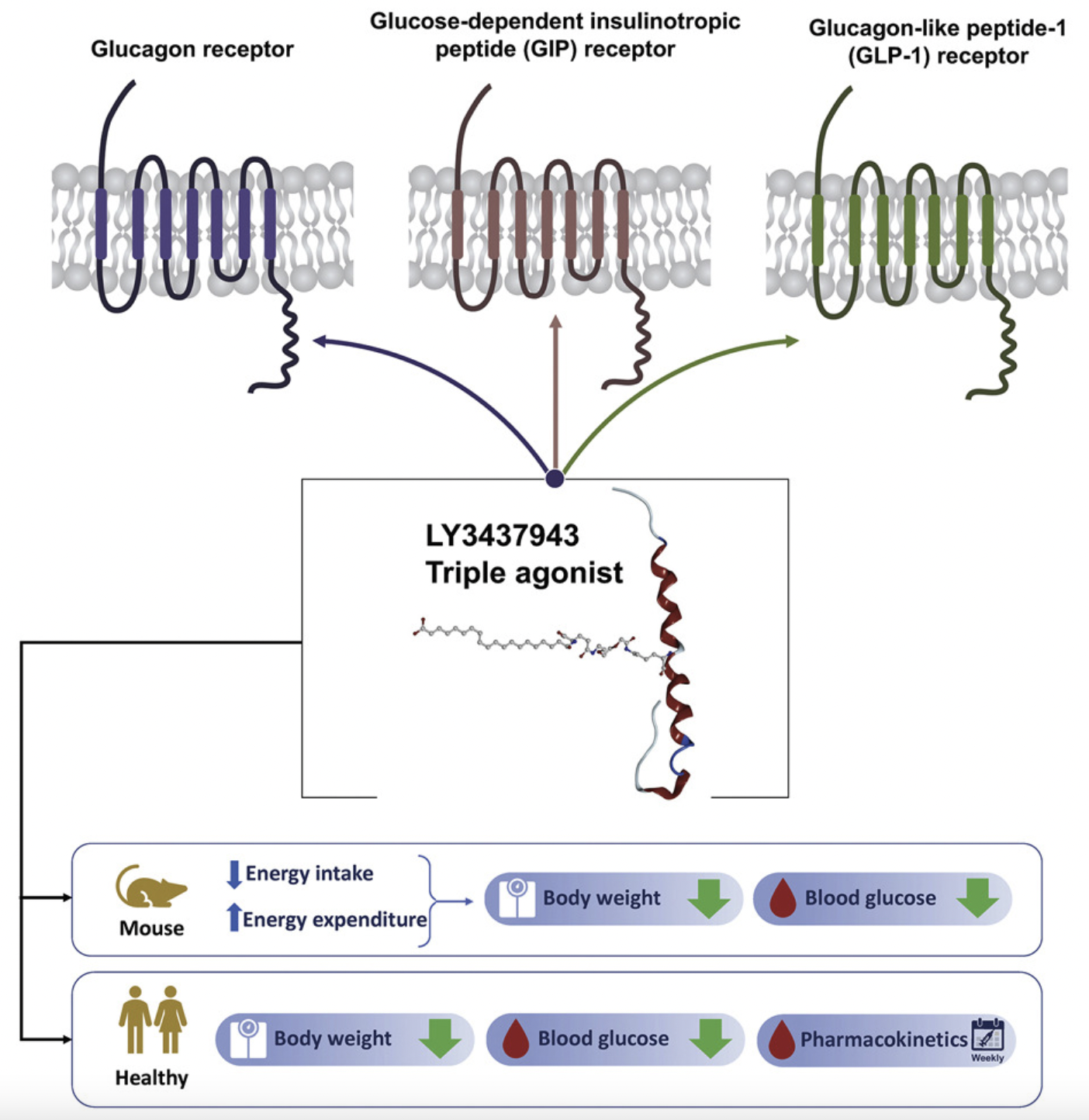
Engineering these drugs is difficult not just because we want to hit many targets in specific ways, but also because measuring the effects of a drug across multiple distinct biological mechanisms is limited by biological toolkits. At Octant, though, we use our Navigator platform to answer tough questions like, “How do our compounds interact with many unique targets simultaneously?” or, “To what extent does a molecule bias signaling towards one pathway over another?” We call our approach to asking these questions “Broad Target Scanning” (BTS) because we are trying to examine how our compounds act broadly on multiple targets, rather than just one.
Another example of how we use BTS is to find and engineer drugs that treat monogenic diseases (sometimes referred to as “rare diseases”). These diseases are caused by a mutation to a particular gene. And in numerous monogenic diseases, many different mutations to that gene can each lead to the same disease. By multiplexing, we can try to engineer molecules molecules that are effective against as many mutations as possible to bring relief to more patients. Instead of building a drug against a known mutation and hoping the drug will be effective for others, we use multiplexing to a-priori identify molecules that are effective against as many of the mutations as possible (Figure 2).
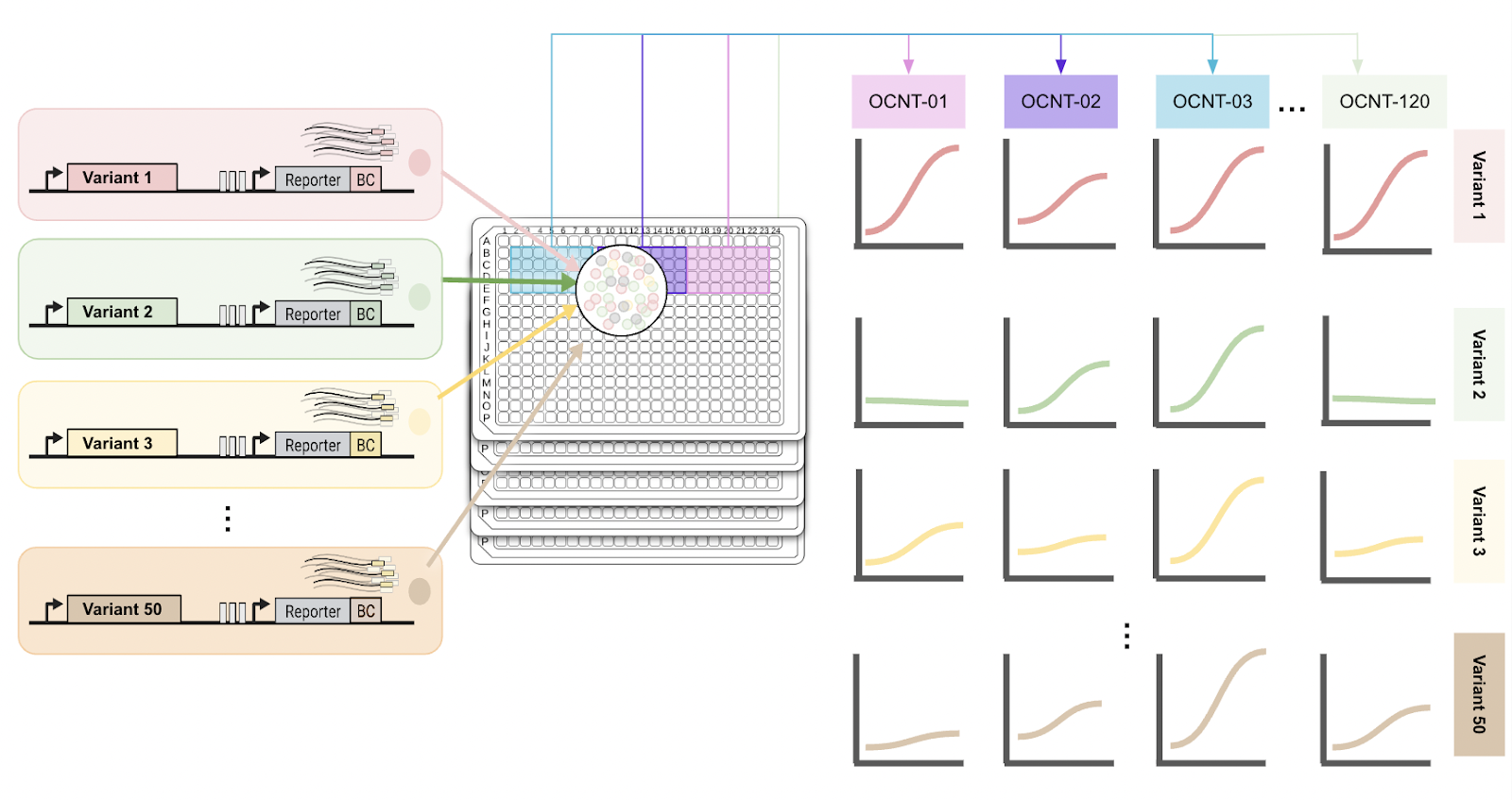
We typically conduct these assays on the order of tens of variants (initially screening the more prevalent variants), but we’ve also built capabilities to test thousands of variants in a method called Deep Mutational Scanning (DMS).
Multiplexing to Enable Deep Mutational Scanning
DMS is a powerful technique to comprehensively investigate how a protein’s structure drives its function. In DMS, cell lines are engineered to each contain a different point mutant of the protein. This is done for every possible mutation, which quickly adds up to thousands of mutants. We pool these cell lines to construct a library of mutants, then perform an assay that quantifies how each mutation at each position on the protein relates to its various properties (i.e. signaling, expression, and substrate selectivity). This information not only drives a deeper understanding of the protein’s genetics and biology, but also can be used to guide rational drug design– focusing our medicinal chemistry towards particular regions of the target (Figure 3). In addition to using it in our own drug programs, we are also deploying DMS technology in a research collaboration with Bristol Myers Squibb.
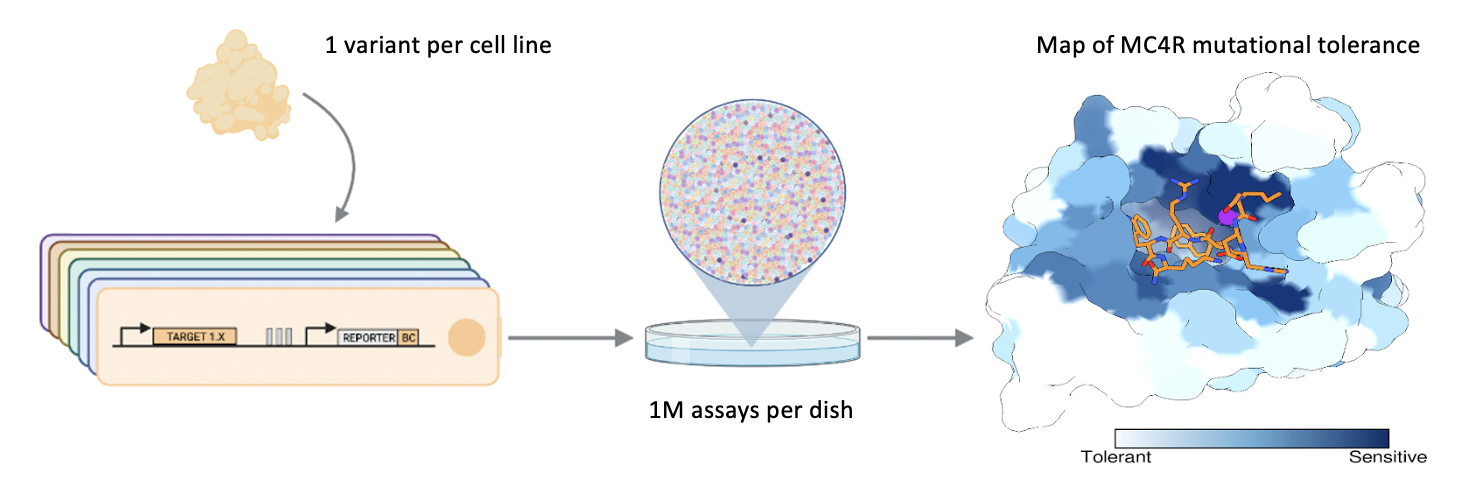
Stay Tuned for Deeper Dives into our Multiplexing Capabilities!
Multiplexing is a powerful tool in our arsenal of synthetic biology capabilities. We use it to build faithful models of cellular mechanisms, guide our engineering of drugs, and inform decisions that affect almost every stage of drug discovery. If you’re interested in diving deeper into each of the applications summarized above, look out for more blog posts from us in the coming months. In each post we’ll showcase a relevant project, highlighting the major challenges and victories we faced, and provide some tips on how scientists can apply some of these approaches to their work.
For the next blog in our series on multiplexing for Cellular Intelligence, check out this post on our Broad Target Scanning approach to developing polypharmacological drugs.
References
1. Dempsey D.A., Klessig D.F. How does the multifaceted plant hormone salicylic acid combat disease in plants and are similar mechanisms utilized in humans? BMC Biol. 2017;15:23. doi: 10.1186/s12915-017-0364-8.
2. Lewin, K. Lilly’s pipeline drug retatrutide sets new threshold for weight loss at medical meeting reveal. Endpoints News. 2023. https://endpts.com/lillys-retatrutide-saw-patients-lose-24-of-weight-at-highest-dose/
3. Coskun T., et al. LY3437943, a novel triple glucagon, GIP, and GLP-1 receptor agonist for glycemic control and weight loss: From discovery to clinical proof of concept. Cell Metab. 2022;1234-1247. doi: 10.1016/j.cmet.2022.07.013.
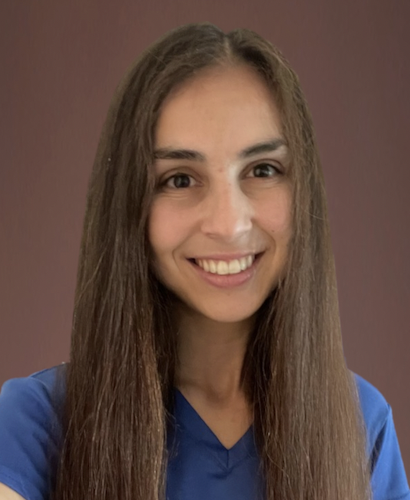